Endogenous calcium mediates seedling growth and fluoride stress tolerance in four bean genotypes
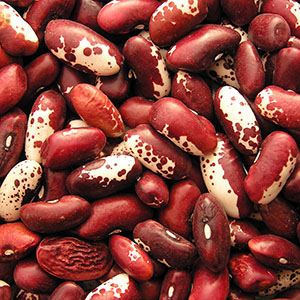
Accepted: 13 June 2022
HTML: 208
All claims expressed in this article are solely those of the authors and do not necessarily represent those of their affiliated organizations, or those of the publisher, the editors and the reviewers. Any product that may be evaluated in this article or claim that may be made by its manufacturer is not guaranteed or endorsed by the publisher.
Fluoride (F) pollution is a global environmental problem representing a severe risk for food and vegetables grown in contaminated soils. Phaseolus vulgaris L. is widely cultivated in arid and semi-arid regions and F-contaminated areas of the world. For that reason, F tolerance during germination and seedling growth was evaluated for four bean genotypes: Borlotto nano (commercial variety) and three African genotypes (Lyamungu 85, Lyamungu 90, and Jesca). Seeds were grown in sand enriched with NaF or KF at three different levels (0, 80, and 200 mg kg–1). NaCl was used as a benchmark to determine the potential effect of different Na levels in the plant. Total F content and mineral accumulation (Na, K, and Ca) in roots and shoots were measured. The translocation factor, growth ratio, and F tolerance index were evaluated to estimate plant-salt response. Germination rate decreased with increased F level. Borlotto was more F sensitive (0% germination with 200 mg kg–1of KF and NaF) than the African genotypes. Under the highest F concentration (200 mg kg–1), F preferentially accumulated in shoots (Jesca 75.7 mg kg–1, Lyamungu 85 100.1 mg kg–1, and Lyamungu 90 115.4 mg kg–1). Ca content in roots was negatively correlated to F absorption, suggesting its antagonistic role to F mobility. Based on these parameters, Jesca and Lyamungu 85 were the most tolerant species, recording a low F uptake and a high Ca content in the root. This study highlighted the central role of Ca as a key secondary messenger in regulating plant growth and development under F stress.
Highlights
- F stress negatively affected bean germination and seedling growth.
- F mainly accumulated in the shoots of bean varieties.
- Ca concentration in the roots played a crucial role in mitigating F accumulation.
How to Cite

This work is licensed under a Creative Commons Attribution-NonCommercial 4.0 International License.
PAGEPress has chosen to apply the Creative Commons Attribution NonCommercial 4.0 International License (CC BY-NC 4.0) to all manuscripts to be published.